Hello! Cool Concepts are back after a couple of months. In this edition, I am exploring a bunch of things that grabbed my attention recently — a little math, a lot on Space, and some daily problems. One of these topics came on a special request from my brother, and I hope he finds it informative. Feel free to skip and skim, and let me know any thoughts or additional information you may have.
Compliant Mechanisms
A mechanism is a mechanical device that transfers or transforms motion, force, or energy. A pair of scissors, pliers, pumps, pistons, nutcrackers, lemon squeezers, etc., are examples of rigid body mechanisms, meaning they have multiple rigid parts attached by movable joints or levers. Mechanisms can be found in automobiles, robotics, hardware equipment, and anything that has moving parts. The main aim of these mechanisms is to generate more output force or energy than what was applied as input. Take a lemon squeezer, for example. The input force you give at one end is increased at the output, enough to squeeze a lemon. If you give the same force to a lemon directly in the same way, you won't be able to squeeze it.
Compliant mechanisms achieve the same kind of transformations using the flexibility of their parts rather than movable joints. Here's an example of a compliant plier mechanism.
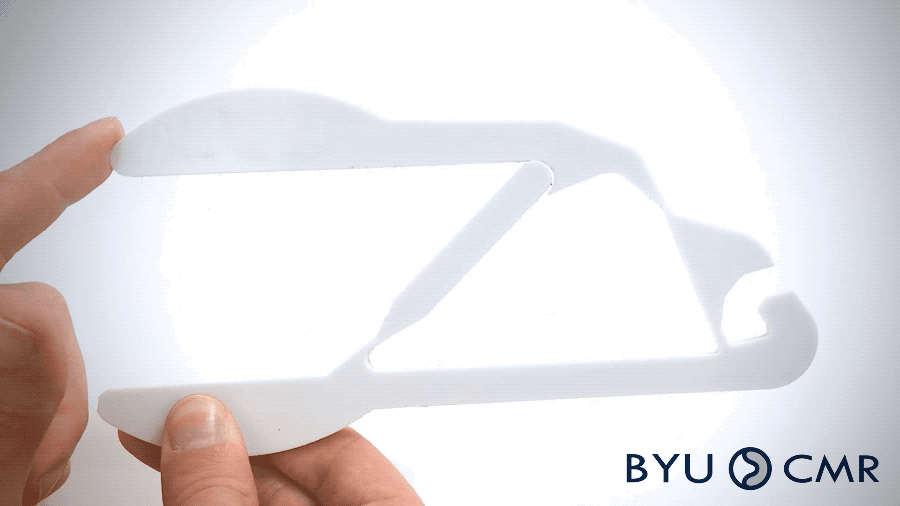
They are a class of machines that transmits force and motion through elastic body deformation. These machines are constructed to use the flexibility of materials to our advantage. The above example is made from a single piece of material, but it generates the same output force as rigid type pliers with joints.
The main advantages of using compliant mechanisms are
- Fewer parts — The number of parts required to accomplish a task is way less in compliant mechanisms than their rigid counterparts. The part count can be reduced by having flexible parts instead of screws, springs, hinges, etc.
- Production processes — Production of compliant mechanisms is significantly easier. As they involve fewer and more flexible parts, they can be manufactured from single planes of materials. For example, the compliant pliers shown above were manufactured from a single polypropylene sheet. Compliant mechanisms can be manufactured using various methods, including machining, laser cutting, 3D printing, water-jet cutting, etc. It also reduces manufacturing costs, especially when they are made in bulk.
- Performance — Compliant mechanisms don't have moveable joints, and even complex mechanisms used by NASA in space have fewer moveable pins, joints, and levers than their rigid counterparts. The mechanisms used in harsh environments such as space may adversely affect the joints and evaporate the lubricants used. The design of compliant mechanisms results in increased performance, reduced friction, and a reduced need for lubrication. Here are some more examples of compliant mechanisms.

Voyager
The Voyagers
Voyager 1 and 2 are spacecraft built by NASA in the 1970s. They are two identical spacecraft made to be sent on different trajectories for the same purpose. Voyager 2 was launched first, and Voyager 1 was launched second, as Voyager 2 was launched on a longer trajectory and would reach Jupiter and Saturn after Voyager 1.
Voyagers 1 and 2 are identical spacecraft. Each is equipped with instruments to conduct ten different experiments. The instruments include television cameras, infrared and ultraviolet sensors, magnetometers, plasma detectors, and cosmic-ray and charged-particle sensors. In addition, the spacecraft radio is used to conduct experiments.
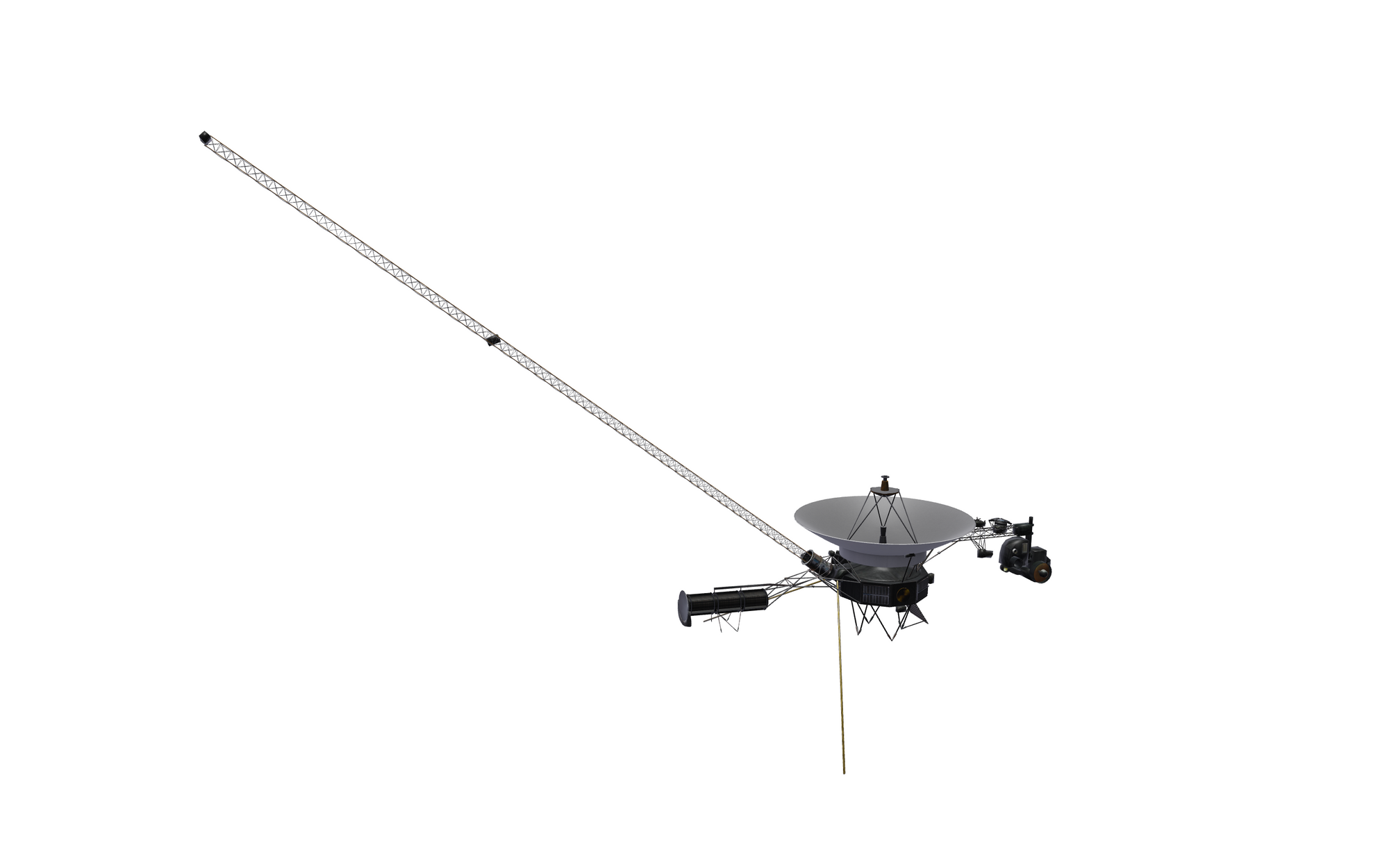
The Voyagers travel too far from the Sun to use solar panels; instead, they were equipped with power sources called radioisotope thermoelectric generators (RTGs). These devices, used on other deep space missions, convert the heat produced from the natural radioactive decay of plutonium into electricity to power the spacecraft instruments, computers, radio, and other systems.
Voyager 1's trajectory, designed to send the spacecraft closely past the giant moon Titan and behind Saturn's rings, bent the spacecraft's path inexorably northward out of the ecliptic plane — the plane in which most of the planets orbit the Sun. Voyager 2 was aimed to fly by Saturn at a point that would automatically send the spacecraft in the direction of Uranus.
Another interesting artifact of the Voyagers is a playable "time-capsule" record called the "Golden Record." The mission scientists decided to include identical records in both the Voyagers. If an extraterrestrial intelligent life form encounters the spacecraft, it intends to show them who we humans are, and it's a message to our extraterrestrial neighbors. The contents of the record were selected for NASA by a committee chaired by Carl Sagan of Cornell University et al. Dr. Sagan and his associates assembled 115 images and a variety of natural sounds, such as those made by surf, wind, thunder, birds, whales, and other animals. They added musical selections from different cultures and eras, spoken greetings from Earth-people in fifty-five languages, and printed messages from President Carter and U.N. Secretary General Waldheim.

The mission
The idea for the project started in the summer of 1965 when it was discovered that it is possible to send a spacecraft to the outer planets of the solar system, using the gravity of the planets themselves to swing the spacecraft from one planet to the next without using external propulsion systems. It was due to the rare alignment of Jupiter, Saturn, Uranus, and Neptune that happens once in 176 years. It was calculated that this would be possible if a spacecraft were launched in the late 1970s.
The Voyagers' original mission was to use this alignment and visit Jupiter and Saturn. As the mission progressed with the successful progression of all its milestones, it was discovered that two additional flybys of Uranus and Neptune were possible. So the mission was expanded to include Uranus and Neptune.
Timeline
Here is a timeline of significant events of the mission. Click on each milestone to view the details.
Summer 1965: A Once-in-a-Lifetime Alignment
Calculations reveal that it was possible to send a spacecraft in the late 1970s to visit all four outer giant planets, using each planet's gravity to propel the spacecraft to the next. This alignment happens once in 176 years.
July 1, 1972: The Project Begins
"Mariner Jupiter/Saturn 1977", the name of the mission before it became Voyager, is approved by NASA, with day-to-day management by the Jet Propulsion Laboratory in Pasadena, California. The original plans commit only to flybys of Jupiter and Saturn and build upon the heritage of earlier Mariner spacecraft that flew by Venus, Mars, and Mercury.
March 1977: Call It 'Voyager'
The Mariner Jupiter/Saturn 1977 project is renamed Voyager.
August 20, 1977: Voyager 2 Launches
Voyager 2 launches from NASA's Kennedy Space Center. It is named Voyager 2 because, even though it launches first, Voyager 2 is planned to reach Jupiter and Saturn after Voyager 1.
September 5, 1977: Voyager 1 Launches
Voyager 1 launches from the Kennedy Space Center.
September 6, 1977: Voyager 1 Views Earth and Moon
Voyager 1 returns the first spacecraft photo of Earth and the Moon.
March 5, 1979: Voyager 1 Encounters Jupiter
Voyager 1 makes its closest approach to Jupiter. Highlights of the encounter include the discoveries of the first active volcanoes spotted beyond Earth at the moon Io, the Jovian ring system, and two moons. Scientists also found that the Great Red Spot is a huge cyclone-like storm, Ganymede (Jupiter's and the solar system's largest moon) has a grooved terrain and that Jupiter has lightning (the first time lightning was detected beyond Earth).
July 9, 1979: Voyager 2 Encounters Jupiter
Voyager 2 makes its closest approach to Jupiter. Highlights of this encounter include the first images of Jupiter's ring system, the discovery of a third moon (Adrastea); a close approach to the moon Europa, and more details about the moon Io.
November 9, 1980: Voyager 1 Encounters Saturn
Voyager 1 makes its closest approach to Saturn's largest moon Titan and Saturn itself. Highlights of the encounter include the discovery of three moons (Atlas, Prometheus, and Pandora), the discovery of ghostly features in Saturn's rings, and the discovery of the first beyond-earth Nitrogen-rich atmosphere on Titan, which is the only thick atmosphere on a natural satellite in the Solar System. After the Saturn encounter, Voyager 1 begins its trip out of the solar system, heading upwards out of the plane of planets.
August 25, 1981: Voyager 2 Encounters Saturn
Voyager 2 makes its closest approach to Saturn. Highlights of this encounter include close encounters with several of Saturn's icy moons, and images of the terrain of the moon Enceladus.
January 24, 1986: Voyager 2 Encounters Uranus
Voyager 2 makes its closest approach to Uranus, the first time the seventh planet from the Sun has been seen up-close. Voyager 2's images reveal 11 new moons, its known moon, Miranda. In this encounter, scientists also learn that Uranus's magnetic field is tilted, so that the poles of its magnetic field are close to the equator, unlike the Earth. Voyager 2 is the first to image Uranus's very dark rings and to detect temperatures around -353ºF, making it the coldest planet in our solar system.
August 25, 1989: Voyager 2 Encounter Neptune
Voyager 2 is the first spacecraft to Neptune up-close and the first to visit four planets beyond Earth. Highlights of the encounter include the discovery of six new moons, the first images of Neptune's rings, the discovery of a huge, counter-clockwise rotating storm in Neptune's southern hemisphere, close-up view of its moon Triton. After the Neptune encounter, Voyager 2 begins its trip out of the solar system, below the plane of the planets. To recap, Voyager 1 has gone upwards after Saturn, and Voyager 2 has gone downwards after Neptune.
October 10 & December 5, 1989: Voyager 2's Cameras Turn Off
Engineers turn off Voyager 2's cameras because they want to use the power, compute memory, and data rate for other instruments that collect key data on the solar wind and interstellar space. The spacecraft will never fly close enough to any astronomical object to take images again.
February 14, 1990: Pale Blue Dot and Friends
At a distance of about 4 billion miles from the sun, Voyager 1 takes the last images of the mission: the "Solar System Family Portrait." It is the only series of images that capture Venus, Earth, Jupiter, Saturn, Uranus, and Neptune arrayed about the sun. Earth is captured as a tiny speck in a beam of scattered sunlight, inspiring Carl Sagan to think about the infamous "Pale Blue Dot" statement about the uniqueness of our home planet (picture and his quote below). After this, the scientists turn off Voyager 1's cameras for the same reasons as Voyager 2.

February 17, 1998: Marathon Runner
Voyager 1 passes the distance of Pioneer 10 to become the farthest human-made object from Earth in space.
December 16, 2004: Voyager 1 Crosses the Shock
Voyager 1 crosses the termination shock, where the solar wind abruptly slows down and heats up as it encounters the interstellar wind.
August 30, 2007: Voyager 2 Crosses the Shock
Voyager 2 crosses the termination shock.
August 13, 2012: The Ultimate Workhorse
Voyager 2 becomes NASA's longest-operating mission, breaking the previous record for continuous operation by Pioneer 6 (12,758 days of operation).
August 25, 2012: The First Human-Made Object in Interstellar Space
Voyager 1 enters interstellar space, passing beyond the heliopause, which is the boundary between our solar bubble and the matter ejected by explosions of other stars. It is the first time a human-made object crosses the threshold of interstellar space. However, the crossing is not confirmed until April 9, 2013.
April 9, 2013: The Ringing of Interstellar Space
Voyager 1 makes the first measurement of the density of the interstellar medium when a wave caused by a coronal mass ejection from the Sun reaches Voyager 1 and causes the plasma in interstellar space to ring. The high pitch of the ringing indicates the density of the plasma surrounding Voyager 1 is more than 40 times higher than previously measured, confirming the spacecraft is in interstellar space and has been since August 25, 2012.
November 5, 2018: NASA's Voyager 2 Probe Enters Interstellar Space
For the second time in history, a human-made object reaches the space between the stars. Voyager 2 exits the heliosphere — the protective bubble of particles and magnetic fields created by the Sun.
Today: The Mission Continues
Both the Voyagers are sending data back to Earth to this day. On May 18, 2022, NASA reported that the readouts from Voyager 1's attitude articulation and control system (AACS) don't reflect what's actually happening onboard. While the spacecraft continues to return science data and otherwise operate as normal, the mission team is searching for the source of a system data issue. When I am writing this, Voyager 1 is at a distance of 14,544,540,603 miles from Earth, while Voyager 2 is at a distance of 12,085,122,504 miles from the Earth. The live distance of both the probes can be viewed via the official website.

Logarithms
Almost all of us have learned logarithms in high school. In high school, we carried a booklet of Clarke's tables to look up the logarithms of numbers for math problems. Having seen so many calculations and graphs represented in linear scale versus logarithmic scale, I have always found it difficult to visualize logarithms in my mind. We have seen that logarithms and exponential functions are related, but I was never able to develop an intuition for it.
When I looked at a video about the Riemann Hypothesis, I unexpectedly stumbled upon an explanation of logarithms. It was so simple that I loathed myself for not understanding it correctly. So, here is an attempt to articulate the logic of logarithms in a way I don't forget.
Definition and examples
A logarithm (l) is the number of times a number (x) must be multiplied together to make another number (y). This sounds very familiar. In simpler terms, a logarithm reverses an exponent, like how division reverses multiplication and how subtraction reverses addition. The notation for this is l = logx(y), read as "log y to the base x."
For example, we know that 23 = 8, meaning if we multiply x = 2 with itself thrice, we get y = 8. Now, the logarithm of 8 is the number of times 2 should be multiplied to arrive at it, which is thrice (l = 3). It is expressed as log28 = 3. The base is the initial number we multiply to get to the final number.
Similarly, to find log5625, all we have to ask is, how many times should I multiply 5 to get 625. The answer is 4 (5 x 5 x 5 x 5 = 625). So log5625 = 4.
Of course, there are more concepts like common logarithms (base = 10), natural logarithms (base = e), change of base rule, etc., but the above method is to grasp the basics.
Timeshare
Timeshare is an interesting concept I heard during a team lunch with my colleagues. A timeshare is a shared ownership model on a property, based on specific periods of time. Timeshares are typically used for vacation homes, condominiums, campsites, and resorts.
Timeshare is a type of fractional ownership where the buyers purchase the right to occupy a unit of real estate over specific periods in a year. For example, buying one week of a timeshare means the buyer owns 1/52 of the property, and a month of a timeshare means the buyer owns 1/12 of the property. Timeshare is most popular in the vacation business, and there are three types of a timeshare.
- Fixed Week — The buyers purchase a fixed week (or weeks) of property ownership every year and own the property for that week (or weeks). But the disadvantage with this plan is that it is not practical to plan a vacation or usage of the property on the exact week every year.
- Floating week — The buyers purchase the duration (week or weeks), but the period is not fixed. This gives some flexibility, but it often results in conflicts between the owners trying to occupy the property at the same time of the year (summer vacation homes, for instance).
- Points — In this type, points are used to represent ownership based on factors such as location, property size, time availability, etc. This type is mainly used by different resort owners on a commercial scale.
Timeshare is not considered a good investment in real estate, and people buy it more for ownership and usage rather than as an investment. However, the concept of shared ownership based on time was interesting and something I had never heard of.
Phantom Traffic Jams
Traffic Jams are an inevitable part of our daily lives. We have all been in a slow-moving traffic jam, which seems to have happened without any apparent reason, such as an accident, a road closure, a stop light, a change in the speed limit, or something else. It is more frustrating knowing that there is nothing evidently causing them. These are known as Phantom Traffic Jams.
A Phantom Traffic Jam occurs due to small changes in the driving habits of individuals on the road. It mainly occurs on highways with heavy traffic when the number of vehicles on the road exceeds a critical density causing dynamic instability. For example, when a driver breaks or slows down without any significant reason on a highway with heavy traffic, it causes a traffic wave that propagates through the rest of the traffic and can continue for miles.
So, the primary cause of this type of phantom traffic jam is the variance in speed of the vehicles on the road with a particular speed limit. Another cause is the improper and unequal spacing of vehicles on the road (typically less than 35 m apart). Check out this simulation of a phantom traffic jam from MIT on a circular route with no blockage. It has been proven with real-life experiments too.
How to avoid it?
Avoiding phantom traffic jams is a collective effort between drivers on the road. Drivers cause a phantom traffic jam when they don't have a good sense of what is happening on the road. Here are some tips to avoid them.
- We should try to break gradually and be alert on the road. Breaking gradually and in advance can significantly reduce phantom traffic jams.
- We should try not to accelerate when the traffic is moving smoothly. One driver accelerating can alert other drivers to brake preemptively, causing a phantom traffic jam to the vehicles behind us. Accelerating unnecessarily can also cause us to brake again further down the road, thus starting another phantom traffic jam.
- We should avoid going too slow or too fast (cutting through the traffic) on roads with considerable amounts of traffic.
- Using navigation apps helps us foresee upcoming traffic to prepare for unforeseen braking or plan for a detour.
- Avoiding phantom traffic is one of the potential advantages of autonomous vehicles. They are mostly equipped with live traffic data and are programmed to maintain minimum distances from the surrounding vehicles and match the average speed of the overall flow. This leads to controlled braking, smooth lane changes, and other optimal maneuvers. In a recent experiment, one autonomous vehicle with 20 human drivers could dampen the traffic wave and reduce phantom traffic.
So, those were the concepts I learned recently. This post marks my 26th week of writing, and I hope it was informative and helpful in some ways. What are some cool things you learned recently? I'd love to know. As always, thanks for reading and your support and feedback over the last 6 months. Peace out!
References
- https://www.compliantmechanisms.byu.edu/about-compliant-mechanisms
- Why Machines that Bend Are Better — Veritasium.
- https://voyager.jpl.nasa.gov/mission/
- https://voyager.jpl.nasa.gov/mission/timeline/#event-a-once-in-a-lifetime-alignment
- A Brief History of Voyager 1 and 2.
- The Riemann Hypothesis, Explained — Quanta Magazine.
- https://math.stackexchange.com/questions/837180/how-to-see-the-logarithm-as-the-inverse-function-of-the-exponential
- https://www.investopedia.com/terms/t/timeshare.asp
- What is phantom traffic and why is it ruining your life? — TedEd.